Degradable Polymers for Biomedical and Biomaterial Applications from Sigma Aldrich
During the last two decades, significant advances have been made in the development of biocompatible and biodegradable materials for biomedical applications, and in the case of the latter category, industrial applications, as well. In the biomedical field, the goal is to develop and characterize artificial materials or, in other words, "spare parts" for use in the human body to measure, restore, and improve physiologic function, and enhance survival and quality of life. Typically, inorganic (metals, ceramics, and glasses) and polymeric (synthetic and natural) materials have been used for such items as artificial heart-valves, (polymeric or carbon-based), synthetic blood-vessels, artificial hips (metallic or ceramic), medical adhesives, sutures, dental composites, and polymers for controlled slow drug delivery. The development of new biocompatible materials includes considerations that go beyond nontoxicity to bioactivity as it relates to interacting with and, in time, being integrated into the biological environment as well as other tailored properties depending on the specific "in vivo" application.
Biomimetics
The parallel field of "biomimetics" may be described as the "abstraction of good design from nature" or, plainly put, the "stealing of ideas from nature". The goal is to make materials for non-biological uses under inspiration from the natural world by combining them with manmade, non-biological devices or processes. This is fast becoming a new research frontier.
Biocompatible Polymers
One area of intense research activity has been the use of biocompatible polymers for controlled drug delivery. It has evolved from the need for prolonged and better control of drug administration. The goal of the controlled release devices is to maintain the drug in the desired therapeutic range with just a single dose. Localized delivery of the drug to a particular body compartment lowers the systemic drug level, reduces the need for follow-up care, preserves medications that are rapidly destroyed by the body, and increases patient comfort and/or improves compliance. In general, release rates are determined by the design of the system and are nearly independent of environmental conditions.
Degradable Polymers
A variety of natural, synthetic, and biosynthetic polymers are bio- and environmentally degradable. A polymer based on the C-C backbone tends to be nonbiodegradable, whereas heteroatom-containing polymer backbones confer biodegradability. Biodegradability can therefore be engineered into polymers by the judicious addition of chemical linkages such as anhydride, ester, or amide bonds, among others. Figure 1 provides a schematic representation of the types of polymer degradation. The mechanism for degradation is by hydrolysis or enzymatic cleavage resulting in a scission of the polymer backbone. Macroorganisms can eat and, sometimes, digest polymers, and also initiate a mechanical, chemical, or enzymatic aging.
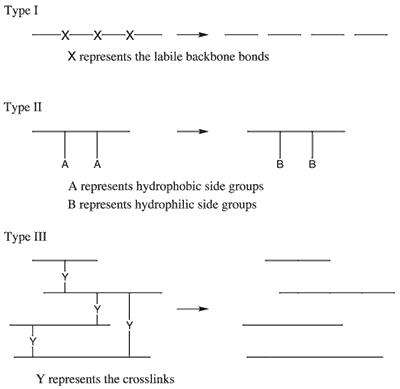
Figure 1. Schematic representation of the types of polymer degradation.
Properties of Degradable Polymers that Make Them Suitable as Biomaterials
Biodegradable polymers with hydrolyzable chemical bonds are being researched extensively for biomedical, pharmaceutical, agricultural, and packaging applications. In order to be used in medical devices and controlled-drug-release applications, the biodegradable polymer must be biocompatible and meet other criteria to be qualified as a biomaterial-processable, sterilizable, and capable of controlled stability or degradation in response to biological conditions. The degradation products often define the biocompatibility of a polymer, not necessarily the polymer itself. Poly(esters) based on polylactide (PLA), polyglycolide (PGA), polycaprolactone (PCL), and their copolymers have been extensively employed as biomaterials. Degradation of these materials yields the corresponding hydroxy acids, making them safe for in vivo use.
Other Bio/Environmentally Degradable Polymers Other bio/environmentally degradable polymers include poly(hydroxyalkanoate)s of the PHB-PHV class, additional poly(ester)s, and natural polymers, particularly, modified poly(saccharide)s, e.g., starch, cellulose, and chitosan.
Chitosan
Chitosan is a technologically important biomaterial. Chitin is the second most abundant natural polymer in the world after cellulose. Upon deacetylation, it yields the novel biomaterial Chitosan, which upon further hydrolysis yields an extremely low molecular weight oligosaccharide (see figure 2). Chitosan possesses a wide range of useful properties. Specifically, it is a biocompatible, antibacterial and environmentally friendly polyelectrolyte, thus lending itself to a variety of applications including water treatment, chromatography, additives for cosmetics, textile treatment for antimicrobial activity, novel fibres for textiles, photographic papers, biodegradable films, biomedical devices, and microcapsule implants for controlled release in drug delivery.
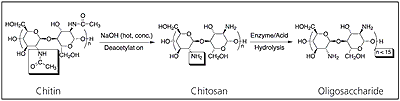
Figure 2. Deacetylation of chitin to form chitosan and hydrolysis to form oligosaccharide.
Poly(ethylene Oxide)Poly(ethylene oxide), PEO, a polymer with the repeat structural unit -CH2CH2O-, has applications in drug delivery. The material known as poly(ethylene glycol), PEG, is in fact PEO but has in addition hydroxyl groups at each end of the molecule. In contrast to high molecular weight PEO, in which the degree of polymerization, n, might range from 103 to 105, the range used most frequently for biomaterials is generally from 12 to 200, that is PEG 600 to PEG 9000, though grades up to 20,000 are commercially available. Key properties that make poly(ethylene oxide) attractive as a biomaterial are biocompatibility, hydrophilicity, and versatility. The simple, water-soluble, linear polymer can be modified by chemical interaction to form water-insoluble but water-swellable hydrogels retaining the desirable properties associated with the ethylene oxide part of the structure.
Poly(ethylene glycol)s first appeared in the U.S. Pharmacopoeia in 1950. Since then they have been used increasingly for a variety of pharmaceutical applications.
Multiblock Copolymers of Poly(ethylene Oxide) and Poly(butylene Terephthalate)
Multiblock copolymers of poly(ethylene oxide) (PEO) and poly(butylene terephthalate) (PBT) are also under development as prosthetic devices and artificial skin and as scaffolds for tissue engineering. These materials are subject to both hydrolysis (via ester bonds) and oxidation (via ether bonds). Degradation rate is influenced by PEO molecular weight and content. Additionally, the copolymer with the highest water uptake degrades most rapidly.
A widely used nondegradable polymer is ethylene-vinyl acetate copolymer. This copolymer displays excellent biocompatibility, physical stability, biological inertness, and processability. In drug delivery application these copolymers usually contain 30-50 weight percent vinyl acetate. Ethylene-vinyl acetate copolymer membrane acts as the rate-limiting barrier for the diffusion of the drug. In the Type II class of degradable polymers, the conversion of the hydrophobic substituents to hydrophilic side groups is a first step in the degradation process. A team of researchers has addressed the problem of fabricating open-pore, biodegradable polymer scaffolds for cell seeding or other tissue engineering applications. The material selected was the tyrosine-derived polycarbonate poly(DTE-co-DT carbonate), in which the pendant group via the tyrosine, an amino acid, is either an ethyl ester (DTE) or free carboxylate (DT). Through alteration of the ratio of DTE to DT, the material’s hydrophobic/hydrophilic balance and rate of in vivo degradation can be manipulated. It was shown that, as DT content increases, pore size decreases, the polymers become more hydrophilic and anionic, and cells attach more readily.
Water Swellable Polymer Networks
Water-swellable polymer networks may function as hydrogels at one end or as superabsorbers at the other extreme. Hydrogels are characterized by the pronounced affinity of their chemical structures for aqueous solutions in which they swell rather than dissolve. Such polymeric networks may range from being mildly absorbing, typically retaining 30 wt. % of water within their structure, to superabsorbing, where they retain many times their weight of aqueous fluids. Several synthetic strategies have been proposed to prepare absorbent polymers including:
· Polyelectrolyte(s) subjected to covalent cross-linking
· Associative polymers consisting of hydrophilic and hydrophobic components ("effective" cross-links through hydrogen bonding)
· Physically interpenetrating polymer networks yielding absorbent polymers of high mechanical strength.
Clearly, these strategies are not mutually exclusive, and efforts have focused on tailoring composite gels which are critically reliant on the balance between polymer-polymer and polymer-solvent interactions under various stimuli including changes in temperature, pH, ionic strength, solvent, concentration, pressure, stress, light intensity, and electric or magnetic fields. Such stimuli-responsive polymers, the so-called smart gels, continue to be the subject of extensive investigation for applications in diverse fields. These applications range from biomedical (controlled drug release, ocular devices, and biomimetics), agricultural (soil additive to conserve water, plant root coating to increase water availability, and seed coating to increase germination rates), and personal care (diapers and adult hygiene products), to industrial (thickener, gelling agent, cable wrap, specialty packaging, tack reduction for natural rubber, and fine coal dewatering).
Absorbent polymers may be of synthetic (petrochemical) origin where the effects of morphology and porosity affects the absorbent properties. Aldrich also offers an extensive selection of polymers of natural (starches, etc.) and semisynthetic (cellulose ethers, etc.) origins for use in the synthesis of multicomponent hydrogels. To aid in designing your application-specific hydrogel, Aldrich offers over 1,500 monomers and a wide selection of cross-linking agents.
<< Home